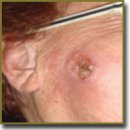
Fluorescent Monitoring of Photodynamic Therapy for Skin Cancer in Clinical Practice
The aim of the investigation was to assess the fluorescent imaging capabilities to monitor photodynamic therapy (PDT) of non-melanoma skin cancer, and study the correlation of PS (photosensitizer) fluorescence value (accumulation and photobleaching rate) and efficacy of the treatment provided.
Materials and Methods. The study was conducted in the Nizhny Novgorod Regional Oncologic Hospital. We analyzed fluorescent images and PDT outcomes in 226 patients with non-melanoma skin carcinomas.
Results. The assessment of short-term treatment results revealed the relationship between PS photobleaching and tumor complete response rate: a complete response was found in 89% cases in complete photobleaching, in 87% cases — in partial photobleaching, and in 81% — in no photobleaching (p>0.05). However, we found no effect of PS accumulation rate on complete response rate. The analysis of long-term results with significant difference (p=0.044) showed tumor recurrence rate in low PS concentration (tumor/norm) to be 9.5%, while in moderate and high concentrations the recurrence rate appeared to be 4.1%. There was revealed the tendency for recurrence rate increase — 10.4% with no PS photobleaching versus 4.4% in PS complete and partial photobleaching (p=0.051). The patients with high accumulation rate and complete PS photobleaching had the best clinical findings, the observation period being from 4 to 40 months.
Conclusion. Fluorescent monitoring enables to maintain noninvasive control of PS accumulation and photobleaching that can contribute to the selection of individual laser exposure parameters. It is reasonable to develop multimodal bioimaging for follow-up real-time monitoring of basic photodynamic reactions and treatment results.
- Foley P., Freeman M., Menter A., Siller G., El-Azhary R.A., Gebauer K., et al. Photodynamic therapy with methyl aminolevulinate for primary nodular basal cell carcinoma: results of two randomized studies. Int J Dermatol 2009 Nov; 48(11): 1236–1245, http://dx.doi.org/10.1111/j.1365-4632.2008.04022.x.
- Agostinis P., Berg K., Cengel K.A., Foster T.H., Girotti A.W., Gollnick S.O., et al. Photodynamic therapy of cancer: an update. CA Cancer J Clin 2011 Jul–Aug; 61(4): 250–281, http://dx.doi.org/10.3322/caac.20114.
- Sokolov V.V., Karpova E.S., Filonenko E.V., Belous T.A., Frank G.A. Successful combination of endoscopic treatment (photodynamic therapy + electrocoagulation) of a patient with early lower third esophageal cancer (14-year clinical observation). Fotodinamicheskaya terapiya i fotodiagnostika 2013; 1: 3–6.
- Allison R.R., Moghissi K. Photodynamic therapy (PDT): PDT mechanisms. Clin Endosc 2013 Jan; 46(1): 24–29, http://dx.doi.org/10.5946/ce.2013.46.1.24.
- Celli J.P., Spring B.Q., Rizvi I., Evans C.L., Samkoe K.S., Verma S., et al. Imaging and photodynamic therapy: mechanisms, monitoring, and optimization. Chem Rev 2010 May; 110(5): 2795–2838, http://dx.doi.org/10.1021/cr900300p.
- Kalugina R.R., Yanvareva I.A., Strel’tsova Yu.A., Gamayunov S.V., Slugarev V.V., Denisenko A.N., et al. Non-invasive monitoring of pathophysiological processes at different photodynamic therapy stages. Sovremennye tehnologii v medicine 2009; 1: 107–110.
- Chissov V.I., Filonenko E.V., Reshetov I.V., Zaytsev A.M., Loshakov V.A., Kurzhupov M.I., et al. Intra-operative fluorescent diagnostics and photodynamic therapy in patients with brain metastatic lesions. Rossiyskiy onkologicheskiy zhurnal 2011; 2: 4–7.
- Kruijt B., van der Ploeg-van den Heuvel A., de Bruijn H.S., Sterenborg H.J., Amelink A., Robinson D.J. Monitoring interstitial m-THPC-PDT in vivo using fluorescence and reflectance spectroscopy. Lasers Surg Med 2009 Nov; 41(9): 653–664, http://dx.doi.org/10.1002/lsm.20845.
- Wang Y., Gu Y., Liao X., Chen R., Ding H. Fluorescence monitoring of a photosensitizer and prediction of the therapeutic effect of photodynamic therapy for port wine stains. Exp Biol Med 2010 Feb; 235(2): 175–180, http://dx.doi.org/10.1258/ebm.2009.009294.
- Brydegaard M., Haj-Hosseini N., Wardell K., Andersson-Engels S. Photobleaching-insensitive fluorescence diagnostics in skin and brain tissue. IEEE Photonics J 2011 Jun; 3(3): 407–421, http://dx.doi.org/10.1109/http://dx.doi.org/10.1109/jphot.2011.2141656.
- Ascencio M., Collinet P., Farine M.O., Mordon S. Protoporphyrin IX fluorescence photobleaching is a useful tool to predict the response of rat ovarian cancer following hexaminolevulinate photodynamic therapy. Lasers Surg Med 2008 Jul; 40(5): 332–341, http://dx.doi.org/10.1002/lsm.20629.
- Shanbhogue A.K., Karnad A.B., Prasad S.R. Tumor response evaluation in oncology: current update. J Comput Assist Tomogr 2010 Jul; 34(4): 479–484, http://dx.doi.org/10.1097/RCT.0b013e3181db2670.
- Common terminology criteria for adverse events (CTCAE). Version 4.03. U.S. Department of Health and Human Services, National Institutes of Health, National Cancer Institute; 2010 June 14.
- Kruijt B., van der Snoek E.M., Sterenborg H.J., Amelink A., Robinson D.J. A dedicated applicator for light delivery and monitoring of PDT of intra-anal intraepithelial neoplasia. Photodiagnosis Photodyn Ther 2010 Mar; 7(1): 3–9, http://dx.doi.org/10.1016/j.pdpdt.2010.01.006.
- Jarvi M.T., Patterson M.S., Wilson B.C. Insights into photodynamic therapy dosimetry: simultaneous singlet oxygen luminescence and photosensitizer photobleaching measurements. Biophys J 2012 Feb; 102(3): 661–671, http://dx.doi.org/10.1016/j.bpj.2011.12.043.
- Xie H., Xie Z., Mousavi M., Bendsoe N., Brydegaard M., Axelsson J., Andersson-Engels S. Design and validation of a fiber optic point probe instrument for therapy guidance and monitoring. J Biomed Opt 2014 Mar; 19(7): 71408, http://dx.doi.org/10.1117/1.JBO.19.7.071408.
- Valdes P.A., Jacobs V.L., Wilson B.C., Leblond F., Roberts D.W., Paulsen K.D. System and methods for wide-field fluorescence imaging during neurosurgery. Opt Lett 2013 Aug; 38(15): 2786–2788, http://dx.doi.org/10.1364/ol.38.002786.
- Sexton K., Davis S.C., McClatchy D., Valdes P.A., Kanick S.C., Paulsen K.D., et al. Pulsed-light imaging for fluorescence guided surgery under normal room lighting. Opt Lett 2013 Sep; 38(17): 3249–3252, http://dx.doi.org/10.1364/OL.38.003249.
- Jacques S.L. How tissue optics affect dosimetry of photodynamic therapy. J Biomed Opt 2010 Sept–Oct; 15(5): 051608, http://dx.doi.org/10.1117/1.3494561.
- Sandell J.L., Zhu T.C. A review of in-vivo optical properties of human tissues and its impact on PDT. J Biophotonics 2011 Nov; 4(11–12): 773–787, http://dx.doi.org/10.1002/jbio.201100062.
- Tyrrell J., Thorn C., Shore A., Campbell S., Curnow A. Oxygen saturation and perfusion changes during dermatological methylaminolaevulinate photodynamic therapy. Br J Dermatol 2011 Dec; 165(6): 1323–1331, http://dx.doi.org/10.1111/j.1365-2133.2011.10554.x.
- Liu B., Farrell T.J., Patterson M.S. A dynamic model for ALA-PDT of skin: simulation of temporal and spatial distributions of ground-state oxygen, photosensitizer and singlet oxygen. Phys Med Biol 2010 Oct; 55(19): 5913–5932, http://dx.doi.org/10.1088/0031-9155/55/19/019.
- Hamdoon Z., Jerjes W., Upile T., Hopper C. Optical coherence tomography-guided photodynamic therapy for skin cancer: case study. Photodiagnosis Photodyn Ther 2011 Mar; 8(1): 49–52, http://dx.doi.org/10.1016/j.pdpdt.2010.08.004.
- Themstrup L., Banzhaf C.A., Mogensen M., Jemec G.B.E. Optical coherence tomography imaging of non-melanoma skin cancer undergoing photodynamic therapy reveals subclinical residual lesions. Photodiagnosis Photodyn Ther 2014 Mar; 11(1): 7–12, http://dx.doi.org/10.1016/j.pdpdt.2013.11.003.
- Mariampillai A., Leung M.K., Jarvi M., Standish B.A., Lee K., Wilson B.C., et al. Optimized speckle variance OCT imaging of microvasculature. Opt Lett 2010 Apr 15; 35(8): 1257–1259, http://dx.doi.org/10.1364/OL.35.001257.
- Zotter S., Pircher M., Torzicky T., Bonesi M., Götzinger E., Leitgeb R.A., Hitzenberger C.K. Visualization of microvasculature by dual-beam phase-resolved Doppler optical coherence tomography. Opt Express 2011; 19(2): 1217–1227, http://dx.doi.org/10.1364/OE.19.001217.
- Ntziachristos V., Razansky D. Molecular imaging by means of multi-spectral optoacoustic tomography (MSOT). Chem Rev 2010; 110(5): 2783–2794, http://dx.doi.org/10.1021/cr9002566.
- Mallidi S., Huang H.C., Liu J.Y., Mensah L., Mai Z., Hasan T. Photoacoustic image guided photodynamic therapy of glioblastoma. Cancer Res 2013 Apr; 73(8 Suppl): 3923, http://dx.doi.org/10.1158/1538-7445.am2013-3923.
- Balu M., Mazhar A., Hayakawa C.K., Mittal R., Krasieva T.B., König K., et al. In vivo multiphoton NADH fluorescence reveals depth-dependent keratinocyte metabolism in human skin. Biophys J 2013 Jan; 104(1): 258–267, http://dx.doi.org/10.1016/j.bpj.2012.11.3809.
- Ulrich M., Klemp M., Darvin M.E., König K., Lademann J., Meinke M.C. In vivo detection of basal cell carcinoma: comparison of a reflectance confocal microscope and a multiphoton tomography. J Biomed Opt 2013 Jun; 18(6): 061229, http://dx.doi.org/10.1117/1.jbo.18.6.061229.
- König K. Hybrid multiphoton multimodal tomography of in vivo human skin. Intravital 2012 Jul; 1(1): 11–26, http://dx.doi.org/10.4161/intv.21938.