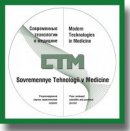
Apoptosis and Cell Proliferation Markers in Inflammatory-Fibroproliferative Diseases of the Vessel Wall (Review)
Apoptosis is the main feature of inflammatory-fibroproliferative disorders of the vessel wall. Studies in animal models have shown that smooth muscle cells (SMCs) cultured from endarterectomy specimens from the affected area proliferate more slowly and display higher apoptotic indices than SMCs derived from the normal vessel wall. Apoptotic cells were found in the destabilized atherosclerotic plaques, as well as in the samples with restenosis of the reconstruction area.
Injury to the vessel wall causes two waves of apoptosis. The first wave is the rapid apoptosis in the media that occurs within a few hours after injury and leads to a marked reduction in the number of vascular wall cells. The second wave of apoptosis occurs much later (from several days to weeks) and is limited by the SMCs within the developing neointima. Up to 14% of the neointimal SMCs undergo apoptosis 20 days after balloon angioplasty. Ligation of the external carotid artery in a rabbit model led to a marked decrease in blood flow in the common carotid artery, which correlated with the increased apoptosis of endothelial cells and SMCs.
Angioplasty-induced death of SMCs is regulated by a redox-sensitive signaling pathway, and topical administration of antioxidants can minimize vascular cell loss. On the whole, studies show that apoptosis is prevalent in vascular lesions, controlling the viability of both inflammatory and vascular cells, determining the cellular composition of the vessel wall. The main markers of apoptosis (Fas, Fas ligand, p53, Bcl-2, Bax) and cell proliferation (toll receptor) have been considered in the current review.
- Pshennikov A.S., Deev R.V. Morphological illustration of alterations in the arterial endothelium in ischemic and reperfusion injuries. Rossiyskiy mediko-biologicheskiy vestnik im. akademika I.P. Pavlova 2018; 26(2): 184–194, https://doi.org/10.23888/pavlovj2018262184-194.
- Kalinin R.E., Suchkov I.A., Pshennikov A.S. The effectiveness of L-arginine in the treatment of atherosclerosis of the arteries of the lower extremities and the prevention of restenosis of the reconstruction zone. Vestnik Ivanovskoy meditsinskoy akademii 2013; 18(2): 18–21.
- D’Arcy M.S. Cell death: a review of the major forms of apoptosis, necrosis and autophagy. Cell Biol Int 2019; 43(6): 582–592, https://doi.org/10.1002/cbin.11137.
- Egorova I.E., Bakhtairova V.I., Suslova A.I. Molecular mechanisms of apoptosis involved in the development of different pathological processes. Innovatsionnye tekhnologii v farmatsii 2019; 6: 107–114.
- Mayboroda A.A. Apoptosis — genes and proteins. Sibirskij medicinskij zhurnal 2013; 3: 130–135.
- Cheng X., Ferrell J.E. Jr. Apoptosis propagates through the cytoplasm as trigger waves. Science 2018; 361(6402): 607–612, https://doi.org/10.1126/science.aah4065.
- Walsh K., Smith R.C., Kim H.S. Vascular cell apoptosis in remodeling, restenosis, and plaque rupture. Circ Res 2000; 87(3): 184–188, https://doi.org/10.1161/01.res.87.3.184.
- Prech M., Marszałek A., Schröder J., Filas V., Lesiak M., Jemielity M., Araszkiewicz A., Grajek S. Apoptosis as a mechanism for the elimination of cardiomyocytes after acute myocardial infarction. Am J Cardiol 2010; 105(9): 1240–1245, https://doi.org/10.1016/j.amjcard.2009.12.039.
- Meng L.B., Shan M.J., Yu Z.M., Lv J., Qi R.M., Guo P., Zhang Y.M., Gong T. Chronic stress: a crucial promoter of cell apoptosis in atherosclerosis. J Int Med Res 2019; 48(1): 300060518814606, https://doi.org/10.1177/0300060518814606.
- Han D.K., Haudenschild C.C., Hong M.K., Tinkle B.T., Leon M.B., Liau G. Evidence for apoptosis in human atherogenesis and in a rat vascular injury model. Am J Pathol 1995; 147(2): 267–277.
- Rivard A., Luo Z., Perlman H., Fabre J.E., Nguyen T., Maillard L., Walsh K. Early cell loss after angioplasty results in a disproportionate decrease in percutaneous gene transfer to the vessel wall. Hum Gene Ther 1999; 10(5): 711–721, https://doi.org/10.1089/10430349950018472.
- Huang P., Hawthorne W.J., Ao P., Angeli G.L., Medbury H.J., Fletcher J.P. Perigraft adventitia and intima remodeling after synthetic patch implantation in sheep carotid artery: role of apoptosis and proliferation. J Vasc Surg 2002; 36(2): 371–378, https://doi.org/10.1067/mva.2002.123749.
- Durham A.L., Speer M.Y., Scatena M., Giachelli C.M., Shanahan C.M. Role of smooth muscle cells in vascular calcification: implications in atherosclerosis and arterial stiffness. Cardiovasc Res 2018; 114(4): 590–600, https://doi.org/10.1093/cvr/cvy010.
- Grootaert M.O.J., Moulis M., Roth L., Martinet W., Vindis C., Bennett M.R., De Meyer G.R.Y. Vascular smooth muscle cell death, autophagy and senescence in atherosclerosis. Cardiovasc Res 2018; 114(4); 622–634, https://doi.org/10.1093/cvr/cvy007.
- Wan L., Dai S.H., Lai S.Q., Liu L.Q., Wang Q., Xu H., Wang W.J., Liu J.C. Apoptosis, proliferation, and morphology during vein graft remodeling in rabbits. Genet Mol Res 2016; 15(4): 371–378, https://doi.org/10.4238/gmr.15048701.
- Ansari M.Z., Swaminathan R. Structure and dynamics at N and C-terminal regions of intrinsically disordered human c-Myc PEST degron reveal a pH-induced transition. Proteins 2020, https://doi.org/10.1002/prot.25880.
- Wei X., Zhang Y., Li C., Ai K., Li K., Li H., Yang J. The evolutionarily conserved MAPK/Erk signaling promotes ancestral T-cell immunity in fish via c-Myc-mediated glycolysis. J Biol Chem 2020; 295(10): 3000–3016, https://doi.org/10.1074/jbc.RA119.012231.
- Date Y., Ito K. Oncogenic RUNX3: a link between p53 deficiency and MYC dysregulation. Mol Cells 2020; 43(2): 176–181, https://doi.org/10.14348/molcells.2019.0285.
- Olivero C.E., Martínez-Terroba E., Zimmer J., Liao C., Tesfaye E., Hooshdaran N., Schofield J.A., Bendor J., Fang D., Simon M.D., Zamudio J.R., Dimitrova N. p53 activates the long noncoding RNA Pvt1b to inhibit Myc and suppress tumorigenesis. Mol Cell 2020; 77(4): 761.e8–774.e8, https://doi.org/10.1016/j.molcel.2019.12.014.
- Lee D.H., Kwon N.E., Lee W.J., Lee M.S., Kim D.J., Kim J.H., Park S.K. Increased O-GlcNAcylation of c-Myc promotes pre-B cell proliferation. Cells 2020; 9(1): E158, https://doi.org/10.3390/cells9010158.
- Yin W., Xia X., Wu M., Yang H., Zhu X., Sun W., Ge M. The impact of BCL-2/MYC protein expression and gene abnormality on primary central nervous system diffuse large B-cell lymphoma. Int J Clin Exp Pathol 2019; 12(6): 2215–2223.
- Steger C.M., Bonaros N., Rieker R.J., Bonatti J., Schachner T. Gene therapy with antisense oligonucleotides silencing c-myc reduces neointima formation and vessel wall thickness in a mouse model of vein graft disease. Exp Mol Pathol 2018; 105(1): 1–9, https://doi.org/10.1016/j.yexmp.2018.05.003.
- Wu Z., Zheng X., Meng L., Fang X., He Y., Li D., Zheng C., Zhang H. α-Tocopherol, especially α-tocopherol phosphate, exerts antiapoptotic and angiogenic effects on rat bone marrow-derived endothelial progenitor cells under high-glucose and hypoxia conditions. J Vasc Surg 2018; 67(4): 1263–1273.e1, https://doi.org/10.1016/j.jvs.2017.02.051.
- Shukla S.K., Sharma S.B., Singh U.R. Pre-treatment with α-tocopherol and terminalia arjuna ameliorates, pro-inflammatory cytokines, cardiac and apoptotic markers in myocardial infracted rats. Redox Rep 2015; 20(2): 49–59, https://doi.org/10.1179/1351000214Y.0000000104.
- Vineetha R.C., Binu P., Arathi P., Nair R.H. L-ascorbic acid and α-tocopherol attenuate arsenic trioxide-induced toxicity in H9c2 cardiomyocytes by the activation of Nrf2 and Bcl2 transcription factors. Toxicol Mech Methods 2018; 28(5): 353–360, https://doi.org/10.1080/15376516.2017.1422578.
- Arias-Álvarez M., García-García R.M., López-Tello J., Rebollar P.G., Gutiérrez-Adán A., Lorenzo P.L. α-Tocopherol modifies the expression of genes related to oxidative stress and apoptosis during in vitro maturation and enhances the developmental competence of rabbit oocytes. Reprod Fertil Dev 2018; 30(12): 1728–1738, https://doi.org/10.1071/rd17525.
- Bozaykut P., Karademir B., Yazgan B., Sozen E., Siow R.C., Mann G.E., Ozer N.K. Effects of vitamin E on peroxisome proliferator-activated receptor γ and nuclear factor-erythroid 2-related factor 2 in hypercholesterolemia-induced atherosclerosis. Free Radic Biol Med 2014; 70: 174–181, https://doi.org/10.1016/j.freeradbiomed.2014.02.017.
- Shibata A., Nakagawa K., Tsuduki T., Miyazawa T. α-Tocopherol suppresses antiangiogenic effect of δ-tocotrienol in human umbilical vein endothelial cells. J Nutr Biochem 2015; 26(4): 345–350, https://doi.org/10.1016/j.jnutbio.2014.11.010.
- Do M.h., Kim S.n., Seo S.Y., Yeo E.J., Kim S.Y. δ-Tocopherol prevents methylglyoxal-induced apoptosis by reducing ROS generation and inhibiting apoptotic signaling cascades in human umbilical vein endothelial cells. Food Funct 2015; 6(5): 1568–1577, https://doi.org/10.1039/c4fo01110d.
- Knapp A.C., Huang J., Starling G., Kiener P.A. Inhibitors of HMG-CoA reductase sensitize human smooth muscle cells to Fas-ligand and cytokine-induced cell death. Atherosclerosis 2000; 152(1): 217–227, https://doi.org/10.1016/s0021-9150(99)00462-1.
- Broniarek I., Jarmuszkiewicz W. Statins and mitochondria. Postepy Biochem 2016; 62(2): 77–84.
- Katsiki N., Tziomalos K., Chatzizisis Y., Elisaf M., Hatzitolios A.I. Effect of HMG-CoA reductase inhibitors on vascular cell apoptosis: beneficial or detrimental? Atherosclerosis 2010; 211(1): 9–14, https://doi.org/10.1016/j.atherosclerosis.2009.12.028.
- Prasad K. Do statins have a role in reduction/prevention of post-PCI restenosis? Cardiovasc Ther 2013; 31(1): 12–26, https://doi.org/10.1111/j.1755-5922.2011.00302.x.
- Erl W., Hristov M., Neureuter M., Yan Z.Q., Hansson G.K., Weber P.C. HMG-CoA reductase inhibitors induce apoptosis in neointima-derived vascular smooth muscle cells. Atherosclerosis 2003; 169(2): 251–258, https://doi.org/10.1016/s0021-9150(03)00201-6.
- Kolben T., Jeschke U., Reimer T., Karsten N., Schmoeckel E., Semmlinger A., Mahner S., Harbeck N., Kolben T.M. Induction of apoptosis in breast cancer cells in vitro by Fas ligand reverse signaling. J Cancer Res Clin Oncol 2018; 144(2): 249–256, https://doi.org/10.1007/s00432-017-2551-y.
- Liu Y., Ren L., Liu W., Xiao Z. MiR-21 regulates the apoptosis of keloid fibroblasts by caspase-8 and the mitochondria-mediated apoptotic signaling pathway via targeting FasL. Biochem Cell Biol 2018; 96(5): 548–555, https://doi.org/10.1139/bcb-2017-0306.
- Mehrbod P., Ande S.R., Alizadeh J., Rahimizadeh S., Shariati A., Malek H., Hashemi M., Glover K.K.M., Sher A.A., Coombs K.M., Ghavami S. The roles of apoptosis, autophagy and unfolded protein response in arbovirus, influenza virus, and HIV infections. Virulence 2019; 10(1): 376–413, https://doi.org/10.1080/21505594.2019.1605803.
- Guégan J.P., Legembre P. Nonapoptotic functions of Fas/CD95 in the immune response. FEBS J 2018; 285(5): 809–827, https://doi.org/10.1111/febs.14292.
- Matter C.M., Chadjichristos C.E., Meier P., von Lukowicz T., Lohmann C., Schuler P.K., Zhang D., Odermatt B., Hofmann E., Brunner T., Kwak B.R., Lüscher T.F. Role of endogenous Fas (CD95/Apo-1) ligand in balloon-induced apoptosis, inflammation, and neointima formation. Circulation 2006; 113(15): 1879–1887, https://doi.org/10.1161/CIRCULATIONAHA.106.611731.
- Fukuo K., Hata S., Suhara T., Nakahashi T., Shinto Y., Tsujimoto Y., Morimoto S., Ogihara T. Nitric oxide induces upregulation of Fas and apoptosis in vascular smooth muscle. Hypertension 1996; 27(3 Pt 2): 823–826, https://doi.org/10.1161/01.hyp.27.3.823.
- Faletti L., Peintner L., Neumann S., Sandler S., Grabinger T., Mac Nelly S., Merfort I., Huang C.H., Tschaharganeh D., Kang T.W., Heinzmann F., D’Artista L., Maurer U., Brunner T., Lowe S., Zender L., Borner C. TNFα sensitizes hepatocytes to FasL-induced apoptosis by NFκB-mediated Fas upregulation. Cell Death Dis 2018; 9(9): 909, https://doi.org/10.1038/s41419-018-0935-9.
- English W.R., Ireland-Zecchini H., Baker A.H., Littlewood T.D., Bennett M.R., Murphy G. Tissue inhibitor of metalloproteinase-3 (TIMP-3) induces Fas dependent apoptosis in human vascular smooth muscle cells. PLoS One 2018; 13(4): e0195116, https://doi.org/10.1371/journal.pone.0195116.
- Imanishi T., McBride J., Ho Q., O’Brien K.D., Schwartz S.M., Han D.K. Expression of cellular FLICE-inhibitory protein in human coronary arteries and in a rat vascular injury model. Am J Pathol 2000; 156(1): 125–137, https://doi.org/10.1016/S0002-9440(10)64712-8.
- Sata M., Perlman H., Muruve D.A., Silver M., Ikebe M., Libermann T.A., Oettgen P., Walsh K. Fas ligand gene transfer to the vessel wall inhibits neointima formation and overrides the adenovirus-mediated T cell response. Proc Natl Acad Sci U S A 1998; 95(3): 1213–1217, https://doi.org/10.1073/pnas.95.3.1213.
- Luo Z., Sata M., Nguyen T. Adenovirus-mediated delivery of Fas ligand inhibits intimal hyperplasia after balloon injury in immunologically primed animals. Circulation 1999; 99(14): 1776–1779, https://doi.org/10.1161/01.cir.99.14.1776.
- Sata M., Sugiura S., Yoshizumi M., Ouchi Y., Hirata Y., Nagai R. Acute and chronic smooth muscle cell apoptosis after mechanical vascular injury can occur independently of the Fas-death pathway. Arterioscler Thromb Vasc Biol 2001; 21(11): 1733–1737, https://doi.org/10.1161/hq1201.098946.
- Anthoney N., Foldi I., Hidalgo A. Toll and toll-like receptor signalling in development. Development 2018; 145(9): dev156018, https://doi.org/10.1242/dev.156018.
- Dishon S., Schumacher-Klinger A., Gilon C., Hoffman A., Nussbaum G. Myristoylation confers oral bioavailability and improves the bioactivity of c(MyD 4-4), a cyclic peptide inhibitor of MyD88. Mol Pharm 2019; 16(4): 1516–1522, https://doi.org/10.1021/acs.molpharmaceut.8b01180.
- Wu K., Zhang H., Fu Y., Zhu Y., Kong L., Chen L., Zhao F., Yu L., Chen X. TLR4/MyD88 signaling determines the metastatic potential of breast cancer cells. Mol Med Rep 2018; 18(3): 3411–3420, https://doi.org/10.3892/mmr.2018.9326.
- Zhu Y., Li Y., Zhang N., Dong G.R. Effect of electroacupuncture preconditioning on myocardial ischemia and expression of TLR 4, MyD 88 and NF-κB mRNAs in “Neiguan” (PC 6) area in rats with myocardial ischemia-reperfusion injury. Zhen Ci Yan Jiu 2018; 43(5): 302–306, https://doi.org/10.13702/j.1000-0607.170308.
- Feng G., Zheng K., Cao T., Zhang J., Lian M., Huang D., Wei C., Gu Z., Feng X. Repeated stimulation by LPS promotes the senescence of DPSCs via TLR4/MyD88-NF-κB-p53/p21 signaling. Cytotechnology 2018; 70(3): 1023–1035, https://doi.org/10.1007/s10616-017-0180-6.
- Cen X., Liu S., Cheng K. The role of toll-like receptor in inflammation and tumor immunity. Front Pharmacol 2018; 9: 878–885, https://doi.org/10.3389/fphar.2018.00878.
- Liu J., Geng F., Sun H., Wang X., Zhang H., Yang Q., Zhang J. Candida albicans induces TLR2/MyD88/NF-κB signaling and inflammation in oral lichen planus-derived keratinocytes. J Infect Dev Ctries 2018; 12(9): 780–786, https://doi.org/10.3855/jidc.8062.
- Cao C., An R., Yu Y., Dai H., Qu Z., Gao M., Wang J. BICP0 negatively regulates TRAF6-mediated NF-κB and interferon activation by promoting K48-linked polyubiquitination of TRAF6. Front Microbiol 2020; 10: 3040, https://doi.org/10.3389/fmicb.2019.03040.
- Li X., Li H., Dong X., Wang X., Zhu J., Cheng Y., Fan P. Expression of NF-κB and TLR-4 is associated with the occurrence, progression and prognosis of esophageal squamous cell carcinoma. Int J Clin Exp Pathol 2018; 11(12): 5850–5859.
- Shao Q., Jiang C., Xia Y., Zhao M., Zhang Q., Jin B., Liu J. Dioscin ameliorates peritoneal fibrosis by inhibiting epithelial-to-mesenchymal transition of human peritoneal mesothelial cells via the TLR4/MyD88/NF-κB signaling pathway. Int J Clin Exp Pathol 2019; 12(3): 867–875.
- Michelsen K.S., Wong M.H., Shah P.K., Zhang W., Yano J., Doherty T.M., Akira S., Rajavashisth T.B., Arditi M. Lack of Toll-like receptor 4 or myeloid differentiation factor 88 reduces atherosclerosis and alters plaque phenotype in mice deficient in apolipoprotein E. Proc Natl Acad Sci U S A 2004; 101: 10679–10684, https://doi.org/10.1073/pnas.0403249101.
- Faghfouri A.H., Zarrin R., Maleki V., Payahoo L., Khajebishak Y. A comprehensive mechanistic review insight into the effects of micronutrients on toll-like receptors functions. Pharmacol Res 2019; 152: 104619, https://doi.org/10.1016/j.phrs.2019.104619.
- Zheng C., Chen J., Chu F., Zhu J., Jin T. Inflammatory role of TLR-MyD88 signaling in multiple sclerosis. Front Mol Neurosci 2020; 12: 314, https://doi.org/10.3389/fnmol.2019.00314.
- Martínez-García M.Á., Ojeda-Ojeda M., Rodríguez-Martín E., Insenser M., Moncayo S., Álvarez-Blasco F., Luque-Ramírez M., Escobar-Morreale H.F. TLR2 and TLR4 surface and gene expression in white blood cells after fasting and oral glucose, lipid and protein challenges: influence of obesity and sex hormones. Biomolecules 2020; 10(1): 111, https://doi.org/10.3390/biom10010111.
- Lee G.L., Chang Y.W., Wu J.Y., Wu M.L., Wu K.K., Yet S.F., Kuo C.C. TLR 2 induces vascular smooth muscle cell migration through cAMP response element-binding protein-mediated interleukin-6 production. Arterioscler Thromb Vasc Biol 2012; 32(11): 2751–2760, https://doi.org/10.1161/atvbaha.112.300302.
- Luo L., Lucas R.M., Liu L., Stow J.L. Signalling, sorting and scaffolding adaptors for toll-like receptors. J Cell Sci 2019; 133(5): jcs239194, https://doi.org/10.1242/jcs.239194.
- Schoneveld A.H., Oude Nijhuis M.M., van Middelaar B., Laman J.D., de Kleijn D.P., Pasterkamp G. Toll-like receptor 2 stimulation induces intimal hyperplasia and atherosclerotic lesion development. Cardiovasc Res 2005; 66(1): 162–169, https://doi.org/10.1016/j.cardiores.2004.12.016.
- Kobayashi N., Suzuki J., Ogawa M., Aoyama N., Komuro I., Izumi Y., Isobe M. Porphyromonasgingivalis promotes neointimal formation after arterial injury through toll-like receptor 2 signaling. Heart Vessels 2014; 29(4): 542–549, https://doi.org/10.1007/s00380-013-0405-3.
- Zhang Y., Xia G., Zhang Y., Liu J., Liu X., Li W., Lv Y., Wei S., Liu J., Quan J. Palmitate induces VSMC apoptosis via toll like receptor (TLR)4/ROS/p53 pathway. Atherosclerosis 2017; 263: 74–81, https://doi.org/10.1007/s00380-013-0405-3.
- Kiechl S., Lorenz E., Reindl M., Wiedermann C.J., Oberhollenzer F., Bonora E., Willeit J., Schwartz D.A. Toll-like receptor 4 polymorphisms and atherogenesis. N Engl J Med 2002; 347(3): 185–192, https://doi.org/10.1056/nejmoa012673.
- Kale J., Osterlund E.J., Andrews D.W. BCL-2 family proteins: changing partners in the dance towards death. Cell Death Differ 2018; 25(1): 65–80, https://doi.org/10.1038/cdd.2017.186.
- Birkinshaw R.W., Czabotar P.E. The BCL-2 family of proteins and mitochondrial outer membrane permeabilisation. Semin Cell Dev Biol 2017; 72: 152–162, https://doi.org/10.1016/j.semcdb.2017.04.001.
- Hockings C., Alsop A.E., Fennell S.C., Lee E.F., Fairlie W.D., Dewson G., Kluck R.M. Mcl-1 and Bcl-xL sequestration of Bak confers differential resistance to BH3-only proteins. Cell Death Differ 2018; 25(4): 721–734, https://doi.org/10.1038/s41418-017-0010-6.
- Edlich F. BCL-2 proteins and apoptosis: recent insights and unknowns. Biochem Biophys Res Commun 2018; 500(1): 26–34, https://doi.org/10.1016/j.bbrc.2017.06.190.
- Walensky L.D. Targeting BAX to drug death directly. Nat Chem Biol 2019; 15(7): 657–665, https://doi.org/10.1038/s41589-019-0306-6.
- Opferman J.T., Kothari A. Anti-apoptotic BCL-2 family members in development. Cell Death Differ 2018; 25(1): 37–45, https://doi.org/10.1038/cdd.2017.170.
- Peña-Blanco A., García-Sáez A.J. Bax, Bak and beyond — mitochondrial performance in apoptosis. FEBS J 2018; 285(3): 416–431, https://doi.org/10.1111/febs.14186.
- Pollman M.J., Hall J.L., Mann M.J., Zhang L., Gibbons G.H. Inhibition of neointimal cell bcl-x expression induces apoptosis and regression of vascular disease. Nat Med 1998; 4(2): 222–227, https://doi.org/10.1038/nm0298-222.
- Zhang J., Huang K., O’Neill K.L., Pang X., Luo X. Bax/Bak activation in the absence of Bid, Bim, Puma, and p53. Cell Death Dis 2016; 7: e2266, https://doi.org/10.1038/cddis.2016.167.
- Bogner C., Kale J., Pogmore J., Chi X., Shamas-Din A., Fradin C., Leber B., Andrews D.W. Allosteric regulation of BH3 proteins in Bcl-xL complexes enables switch-like activation of Bax. Mol Cell 2020; 7(4): 901–912.e9, https://doi.org/10.1016/j.molcel.2019.12.025.
- Borrás C., Mas-Bargues C., Román-Domínguez A., Sanz-Ros J., Gimeno-Mallench L., Inglés M., Gambini J., Viña J. Bcl-xL, a mitochondrial protein involved in successful aging: from C. elegans to human centenarians. Int J Mol Sci 2020; 21(2): 418, https://doi.org/10.3390/ijms21020418.
- Chung S., Roy A.K., Webster T.J. Selenium nanoparticle protection of fibroblast stress: activation of ATF4 and Bcl-xL expression. Int J Nanomedicine 2019; 14: 9995–10007, https://doi.org/10.2147/ijn.s172236.
- Gupta A., Shah K., Oza M.J., Behl T. Reactivation of p53 gene by MDM2 inhibitors: a novel therapy for cancer treatment. Biomed Pharmacother 2019; 109: 484–492, https://doi.org/10.1016/j.biopha.2018.10.155.
- Zhang L., Gao W., Keohavong P. Analysis of mutations in K-ras and p53 genes in sputum and plasma samples. Methods Mol Biol 2020; 2102: 373–394, https://doi.org/10.1007/978-1-0716-0223-2_22.
- Tzovaras A.A., Gentimi F., Nikolaou M. Tumor protein p53 gene and cardiovascular disease. Angiology 2018; 69(8): 736–737, https://doi.org/10.1177/0003319718772412.
- Miyashita T., Reed J.C. Tumor suppressor p53 is a direct transcriptional activator of the human bax gene. Cell 1995; 80(2): 293–299, https://doi.org/10.1016/0092-8674(95)90412-3.
- Wu D., Prives C. Relevance of the p53-MDM2 axis to aging. Cell Death Differ 2018; 25(1): 169–179, https://doi.org/10.1038/cdd.2017.187.
- Wurz R.P., Cee V.J. Targeted degradation of mdm2 as a new approach to improve the efficacy of mdm2-p53 inhibitors. Med Chem 2019; 62(2): 445–447, https://doi.org/10.1021/acs.jmedchem.8b01945.
- Tanaka K., Zou J.P., Takeda K., Ferrans V.J., Sandford G.R., Johnson T.M., Finkel T., Epstein S.E. Effects of human cytomegalovirus immediate-early proteins on p53-mediated apoptosis in coronary artery smooth muscle cells. Circulation 1999; 99(13): 1656–1659, https://doi.org/10.1161/01.cir.99.13.1656.
- Guevara N.V., Kim H.S., Antonova E.I., Chan L. The absence of p53 accelerates atherosclerosis by increasing cell proliferation in vivo. Nat Med 1999; 5(3): 335–339, https://doi.org/10.1038/6585.
- Jacob T., Hingorani A., Ascher E. p53 gene therapy modulates signal transduction in the apoptotic and cell cycle pathways downregulating neointimal hyperplasia. Vasc Endovascular Surg 2012; 46(1): 45–53, https://doi.org/10.1177/1538574411422277.
- Gerber H.P., Dixit V., Ferrara N. Vascular endothelial growth factor induces expression of the antiapoptotic proteins Bcl-2 and A1 in vascular endothelial cells. J Biol Chem 1998; 273(21): 13313–13316, https://doi.org/10.1074/jbc.273.21.13313.
- Svetozarskiy N.L., Artifeksova A.A., Svetozarskiy S.N. Growth promoting factor of endothelium of vessels: biological properties and practical value (literature review). Meditsina i obrazovanie v Sibiri 2015; 5: 24–32.
- Duran X., Vilahur G., Badimon L. Exogenous in vivo NO-donor treatment preserves p53 levels and protects vascular cells from apoptosis. Atherosclerosis 2009; 205(1): 101–106, https://doi.org/10.1016/j.atherosclerosis.2008.11.016.
- Spiguel L.R., Chandiwal A., Vosicky J.E., Weichselbaum R.R., Skelly C.L. Concomitant proliferation and caspase-3 mediated apoptosis in response to low shear stress and balloon injury. J Surg Res 2010; 161(1): 146–155, https://doi.org/10.1016/j.jss.2008.11.001.
- Lee M., Rey K., Besler K., Wang C., Choy J. Immunobiology of nitric oxide and regulation of inducible nitric oxide synthase. Results Probl Cell Differ 2017; 62: 181–207, https://doi.org/10.1007/978-3-319-54090-0_8.
- Boyd C.S., Cadenas E. Nitric oxide and cell signaling pathways in mitochondrial-dependent apoptosis. Biol Chem 2002; 383(3–4): 411–423, https://doi.org/10.1515/bc.2002.045.
- Takahashi А., Masuda А., Sun M., Centonze V.E. Oxidative stress-induced apoptosis is associated with alterations in mitochondrial caspase activity and Bcl-2-dependent alterations in mitochondrial pH (pHm). Brain Res Bull 2004; 62(6): 497–504, https://doi.org/10.1016/j.brainresbull.2003.07.009.
- Chae I.H., Park K.W., Kim H.S., Oh B.H. Nitric oxide-induced apoptosis is mediated by Bax/Bcl-2 gene expression, transition of cytochrome c, and activation of caspase-3 in rat vascular smooth muscle cells. Clin Chim Acta 2004; 341(1–2): 83–91, https://doi.org/10.1016/j.cccn.2003.11.009.
- Choi B.M., Pae H.O., Jang S.I., Kim Y.M., Chung H.T. Nitric oxide as a pro-apoptotic as well as anti-apoptotic modulator. J Biochem Mol Biol 2002; 35(1): 116–126, https://doi.org/10.5483/bmbrep.2002.35.1.116.
- Chung Н.T., Pae H.O., Choi B.M., Billiar T.R., Kim Y.M. Nitric oxide as a bioregulator of apoptosis. Biochem Biophys Res Commun 2001; 282(5): 1075–1079, https://doi.org/10.1006/bbrc.2001.4670.
- Dubey M., Nagarkoti S., Awasthi D., Singh A.K., Chandra T., Kumaravelu J., Barthwal M.K., Dikshit M. Nitric oxide-mediated apoptosis of neutrophils through caspase-8 and caspase-3-dependent mechanism. Cell Death Dis 2016; 7(9): е2348, https://doi.org/10.1038/cddis.2016.248.
- Li X., Shang B., Li Y.N., Shi Y., Shao C. IFNγ and TNFα synergistically induce apoptosis of mesenchymal stem/stromal cells via the induction of nitric oxide. Stem Cell Res Ther 2019; 10(1): 18–24, https://doi.org/10.1186/s13287-018-1102-z.
- Zhang N., Diao Y., Hua R., Wang J., Han S., Li J., Yin Y. Nitric oxide-mediated pathways and its role in the degenerative diseases. Front Biosci (Landmark Ed) 2017; 22: 824–834, https://doi.org/10.2741/4519.