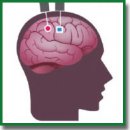
Нейрокомпьютерные интерфейсы с корковыми имплантатами для компенсации двигательной и коммуникативной функций: обзор последних достижений
Нейрокомпьютерные интерфейсы позволяют осуществлять обмен данными между мозгом и внешним техническим устройством в обход мышечной системы. Клинические исследования инвазивных нейроинтерфейсных технологий проводятся уже более 20 лет. Постоянно совершенствуются подходы к обработке нейронального сигнала для повышения качества управления внешними техническими устройствами. На сегодняшний день нейрокомпьютерные интерфейсы с внутрикорковыми датчиками позволяют полностью парализованным пациентам управлять роботизированными конечностями для самообслуживания, пользоваться компьютером или планшетом, набирать текст и воспроизводить речь с оптимальной скоростью. Исследования инвазивных нейроинтерфейсов предоставляют новые данные о функционировании центральной нервной системы. В последние годы в этой области ежегодно происходят прорывные открытия.
В данном обзоре проанализированы результаты клинических испытаний нейрокомпьютерных интерфейсов с внутрикорковыми датчиками, представлены информация об этапах развития данной технологии и основные достижения, связанные с ней.
- Mokienko O.A., Lyukmanov R.Kh., Bobrov P.D., Suponeva N.A., Piradov M.A. Brain–computer interfaces for upper limb motor recovery after stroke: current status and development prospects (review). Sovremennye tehnologii v medicine 2023; 15(6): 63, https://doi.org/10.17691/stm2023.15.6.07.
- Zhao Z.P., Nie C., Jiang C.T., Cao S.H., Tian K.X., Yu S., Gu J.W. Modulating brain activity with invasive brain-computer interface: a narrative review. Brain Sci 2023; 13(1): 134, https://doi.org/10.3390/brainsci13010134.
- Carvalho R., Dias N., Cerqueira J.J. Brain-machine interface of upper limb recovery in stroke patients rehabilitation: a systematic review. Physiother Res Int 2019; 24(2): e1764, https://doi.org/10.1002/pri.1764.
- Baniqued P.D.E., Stanyer E.C., Awais M., Alazmani A., Jackson A.E., Mon-Williams M.A., Mushtaq F., Holt R.J. Brain-computer interface robotics for hand rehabilitation after stroke: a systematic review. J Neuroeng Rehabil 2021; 18(1): 15, https://doi.org/10.1186/s12984-021-00820-8.
- Fu J., Chen S., Jia J. Sensorimotor rhythm-based brain-computer interfaces for motor tasks used in hand upper extremity rehabilitation after stroke: a systematic review. Brain Sci 2022; 13(1): 56, https://doi.org/10.3390/brainsci13010056.
- Bai Z., Fong K.N.K., Zhang J.J., Chan J., Ting K.H. Immediate and long-term effects of BCI-based rehabilitation of the upper extremity after stroke: a systematic review and meta-analysis. J Neuroeng Rehabil 2020; 17(1): 57, https://doi.org/10.1186/s12984-020-00686-2.
- Kruse A., Suica Z., Taeymans J., Schuster-Amft C. Effect of brain-computer interface training based on non-invasive electroencephalography using motor imagery on functional recovery after stroke — a systematic review and meta-analysis. BMC Neurol 2020; 20(1): 385, https://doi.org/10.1186/s12883-020-01960-5.
- Yang W., Zhang X., Li Z., Zhang Q., Xue C., Huai Y. The effect of brain-computer interface training on rehabilitation of upper limb dysfunction after stroke: a meta-analysis of randomized controlled trials. Front Neurosci 2021; 15: 766879, https://doi.org/10.3389/fnins.2021.766879.
- Mansour S., Ang K.K., Nair K.P.S., Phua K.S., Arvaneh M. Efficacy of brain-computer interface and the impact of its design characteristics on poststroke upper-limb rehabilitation: a systematic review and meta-analysis of randomized controlled trials. Clin EEG Neurosci 2022; 53(1): 79–90, https://doi.org/10.1177/15500594211009065.
- Peng Y., Wang J., Liu Z., Zhong L., Wen X., Wang P., Gong X., Liu H. The application of brain-computer interface in upper limb dysfunction after stroke: a systematic review and meta-analysis of randomized controlled trials. Front Hum Neurosci 2022; 16: 798883, https://doi.org/10.3389/fnhum.2022.798883.
- Nojima I., Sugata H., Takeuchi H., Mima T. Brain-computer interface training based on brain activity can induce motor recovery in patients with stroke: a meta-analysis. Neurorehabil Neural Repair 2022; 36(2): 83–96, https://doi.org/10.1177/15459683211062895.
- Xie Y.L., Yang Y.X., Jiang H., Duan X.Y., Gu L.J., Qing W., Zhang B., Wang Y.X. Brain-machine interface-based training for improving upper extremity function after stroke: a meta-analysis of randomized controlled trials. Front Neurosci 2022; 16: 949575, https://doi.org/10.3389/fnins.2022.949575.
- Shou Y.Z., Wang X.H., Yang G.F. Verum versus Sham brain-computer interface on upper limb function recovery after stroke: a systematic review and meta-analysis of randomized controlled trials. Medicine (Baltimore) 2023; 102(26): e34148, https://doi.org/10.1097/md.0000000000034148.
- Frolov A.A., Mokienko O., Lyukmanov R., Biryukova E., Kotov S., Turbina L., Nadareyshvily G., Bushkova Y. Post-stroke rehabilitation training with a motor-imagery-based brain-computer interface (BCI)-controlled hand exoskeleton: a randomized controlled multicenter trial. Front Neurosci 2017; 11: 400, https://doi.org/10.3389/fnins.2017.00400.
- Lyukmanov R.K., Isaev M.R., Mokienko O.A., Bobrov P.D., Ikonnikova E.S., Cherkasova A.N., Suponeva N.A. Brain–computer interface using functional near-infrared spectroscopy for post-stroke motor rehabilitation: case series. Ann Clin Exp Neurol 2023; 17(4): 82–88, https://doi.org/10.54101/ACEN.2023.4.10.
- Dong Y., Wang S., Huang Q., Berg RW., Li G., He J. Neural decoding for intracortical brain–computer interfaces. Cyborg Bionic Syst 2023; 4: 0044, https://doi.org/10.34133/cbsystems.0044.
- Vansteensel M.J., Pels E.G.M., Bleichner M.G., Branco M.P., Denison T., Freudenburg Z.V., Gosselaar P., Leinders S., Ottens T.H., Van Den Boom M.A., Van Rijen P.C., Aarnoutse E.J., Ramsey N.F. Fully implanted brain–computer interface in a locked-in patient with ALS. N Engl J Med 2016; 375(21): 2060–2066, https://doi.org/10.1056/nejmoa1608085.
- Hochberg L.R., Serruya M.D., Friehs G.M., Mukand J.A., Saleh M., Caplan A.H., Branner A., Chen D., Penn R.D., Donoghue J.P. Neuronal ensemble control of prosthetic devices by a human with tetraplegia. Nature 2006; 442(7099): 164–171, https://doi.org/10.1038/nature04970.
- Hochberg L.R., Bacher D., Jarosiewicz B., Masse N.Y., Simeral J.D., Vogel J., Haddadin S., Liu J., Cash S.S., van der Smagt P., Donoghue J.P. Reach and grasp by people with tetraplegia using a neurally controlled robotic arm. Nature 2012; 485(7398): 372–375, https://doi.org/10.1038/nature11076.
- Collinger J.L., Wodlinger B., Downey J.E., Wang W., Tyler-Kabara E.C., Weber D.J., McMorland A.J.C., Velliste M., Boninger M.L., Schwartz A.B. High-performance neuroprosthetic control by an individual with tetraplegia. Lancet 2013; 381(9866): 557–564, https://doi.org/10.1016/s0140-6736(12)61816-9.
- Anumanchipalli G.K., Chartier J., Chang E.F. Speech synthesis from neural decoding of spoken sentences. Nature 2019; 568(7753): 493–498, https://doi.org/10.1038/s41586-019-1119-1.
- Willett F.R., Avansino D.T., Hochberg L.R., Henderson J.M., Shenoy K.V. High-performance brain-to-text communication via handwriting. Nature 2021; 593(7858): 249–254, https://doi.org/10.1038/s41586-021-03506-2.
- Flesher S.N., Downey J.E., Weiss J.M., Hughes C.L., Herrera A.J., Tyler-Kabara E.C., Boninger M.L., Collinger J.L., Gaunt R.A. A brain–computer interface that evokes tactile sensations improves robotic arm control. Science 2021; 372(6544): 831–836, https://doi.org/10.1126/science.abd0380.
- Willett F.R., Kunz E.M., Fan C., Avansino D.T., Wilson G.H., Choi E.Y., Kamdar F., Glasser M.F., Hochberg L.R., Druckmann S., Shenoy K.V., Henderson J.M. A high-performance speech neuroprosthesis. Nature 2023; 620(7976): 1031–1036, https://doi.org/10.1038/s41586-023-06377-x.
- Aflalo T., Kellis S., Klaes C., Lee B., Shi Y., Pejsa K., Shanfield K., Hayes-Jackson S., Aisen M., Heck C., Liu C., Andersen R.A. Neurophysiology. Decoding motor imagery from the posterior parietal cortex of a tetraplegic human. Science 2015; 348(6237): 906–910, https://doi.org/10.1126/science.aaa5417.
- Metzger S.L., Littlejohn K.T., Silva A.B., Moses D.A., Seaton M.P., Wang R., Dougherty M.E., Liu J.R., Wu P., Berger M.A., Zhuravleva I., Tu-Chan A., Ganguly K., Anumanchipalli G.K., Chang E.F. A high-performance neuroprosthesis for speech decoding and avatar control. Nature 2023; 620(7976): 1037–1046, https://doi.org/10.1038/s41586-023-06443-4.
- Ajiboye A.B., Willett F.R., Young D.R., Memberg W.D., Murphy B.A., Miller J.P., Walter B.L., Sweet J.A., Hoyen H.A., Keith M.W., Peckham P.H., Simeral J.D., Donoghue J.P., Hochberg L.R., Kirsch R.F. Restoration of reaching and grasping movements through brain-controlled muscle stimulation in a person with tetraplegia: a proof-of-concept demonstration. Lancet 2017; 389(10081): 1821–1830, https://doi.org/10.1016/s0140-6736(17)30601-3.
- Wang W., Collinger J.L., Degenhart A.D., Tyler-Kabara E.C., Schwartz A.B., Moran D.W., Weber D.J., Wodlinger B., Vinjamuri R.K., Ashmore R.C., Kelly J.W., Boninger M.L. An electrocorticographic brain interface in an individual with tetraplegia. PloS One 2013; 8(2): e55344, https://doi.org/10.1371/journal.pone.0055344.
- Davis K.C., Meschede-Krasa B., Cajigas I., Prins N.W., Alver C., Gallo S., Bhatia S., Abel J.H., Naeem J.A., Fisher L., Raza F., Rifai W.R., Morrison M., Ivan M.E., Brown E.N., Jagid J.R., Prasad A. Design-development of an at-home modular brain–computer interface (BCI) platform in a case study of cervical spinal cord injury. J Neuroeng Rehabil 2022; 19(1): 53, https://doi.org/10.1186/s12984-022-01026-2.
- Oxley T.J., Yoo P.E., Rind G.S., Ronayne S.M., Lee C.M.S., Bird C., Hampshire V., Sharma R.P., Morokoff A., Williams D.L., MacIsaac C., Howard M.E., Irving L., Vrljic I., Williams C., John S.E., Weissenborn F., Dazenko M., Balabanski A.H., Friedenberg D., Burkitt A.N., Wong Y.T., Drummond K.J., Desmond P., Weber D., Denison T., Hochberg L.R., Mathers S., O’Brien T.J., May C.N., Mocco J., Grayden D.B., Campbell B.C.V., Mitchell P., Opie N.L. Motor neuroprosthesis implanted with neurointerventional surgery improves capacity for activities of daily living tasks in severe paralysis: first in-human experience. J Neurointerv Surg 2021; 13(2): 102–108, https://doi.org/10.1136/neurintsurg-2020-016862.
- Mitchell P., Lee S.C.M., Yoo P.E., Morokoff A., Sharma R.P., Williams D.L., MacIsaac C., Howard M.E., Irving L., Vrljic I., Williams C., Bush S., Balabanski A.H., Drummond K.J., Desmond P., Weber D., Denison T., Mathers S., O’Brien T.J., Mocco J., Grayden D.B., Liebeskind D.S., Opie N.L., Oxley T.J., Campbell B.C.V. Assessment of safety of a fully implanted endovascular brain-computer interface for severe paralysis in 4 patients: the stentrode with thought-controlled digital switch (SWITCH) study. JAMA Neurol 2023; 80(3): 270–278, https://doi.org/10.1001/jamaneurol.2022.4847.
- Saha S., Mamun K.A., Ahmed K., Mostafa R., Naik G.R., Darvishi S., Khandoker A.H., Baumert M. Progress in brain computer interface: challenges and opportunities. Front Syst Neurosci 2021; 15: 578875, https://doi.org/10.3389/fnsys.2021.578875.
- Stavisky S.D., Willett F.R., Wilson G.H., Murphy B.A., Rezaii P., Avansino D.T., Memberg W.D., Miller J.P., Kirsch R.F., Hochberg L.R., Ajiboye A.B., Druckmann S., Shenoy K.V., Henderson J.M. Neural ensemble dynamics in dorsal motor cortex during speech in people with paralysis. ELife 2019; 8: e46015, https://doi.org/10.7554/elife.46015.
- Stavisky S.D., Willett F.R., Avansino D.T., Hochberg L.R., Shenoy K.V., Henderson J.M. Speech-related dorsal motor cortex activity does not interfere with iBCI cursor control. J Neural Eng 2020; 17(1): 016049, https://doi.org/10.1088/1741-2552/ab5b72.
- Humphrey D.R., Schmidt E.M., Thompson W.D. Predicting measures of motor performance from multiple cortical spike trains. Science 1970; 170(3959): 758–762, https://doi.org/10.1126/science.170.3959.758.
- Lilly J.C. Instantaneous relations between the activities of closely spaced zones on the cerebral cortex; electrical figures during responses and spontaneous activity. Am J Physiol 1954; 176(3): 493–504, https://doi.org/10.1152/ajplegacy.1954.176.3.493.
- Fetz E.E. Operant conditioning of cortical unit activity. Science 1969; 163(3870): 955–958, https://doi.org/10.1126/science.163.3870.955.
- Schmidt E.M. Single neuron recording from motor cortex as a possible source of signals for control of external devices. Ann Biomed Eng 1980; 8(4–6): 339–349, https://doi.org/10.1007/bf02363437.
- Ifft P.J., Shokur S., Li Z., Lebedev M.A., Nicolelis M.A.L. A brain-machine interface enables bimanual arm movements in monkeys. Sci Transl Med 2013; 5(210): 210ra154, https://doi.org/10.1126/scitranslmed.3006159.
- Chapin J.K., Moxon K.A., Markowitz R.S., Nicolelis M.A.L. Real-time control of a robot arm using simultaneously recorded neurons in the motor cortex. Nat Neurosci 1999; 2(7): 664–670, https://doi.org/10.1038/10223.
- Taylor D.M., Tillery S.I.H., Schwartz A.B. Direct cortical control of 3D neuroprosthetic devices. Science 2002; 296(5574): 1829–1832, https://doi.org/10.1126/science.1070291.
- Wessberg J., Stambaugh C.R., Kralik J.D., Beck P.D., Laubach M., Chapin J.K., Kim J., Biggs S.J., Srinivasan M.A., Nicolelis M.A.L. Real-time prediction of hand trajectory by ensembles of cortical neurons in primates. Nature 2000; 408(6810): 361–365, https://doi.org/10.1038/35042582.
- Carmena J.M., Lebedev M.A., Crist R.E., O’Doherty J.E., Santucci D.M., Dimitrov D.F., Patil P.G., Henriquez C.S., Nicolelis M.A.L. Learning to control a brain–machine interface for reaching and grasping by primates. PLoS Biol 2003; 1(2): E42, https://doi.org/10.1371/journal.pbio.0000042.
- Lebedev M.A., Carmena J.M., O’Doherty J.E., Zacksenhouse M., Henriquez C.S., Principe J.C., Nicolelis M.A.L. Cortical ensemble adaptation to represent velocity of an artificial actuator controlled by a brain-machine interface. J Neurosci 2005; 25(19): 4681–4693, https://doi.org/10.1523/jneurosci.4088-04.2005.
- O’Doherty J.E., Lebedev M.A., Hanson T.L., Fitzsimmons N.A., Nicolelis M.A.L. A brain-machine interface instructed by direct intracortical microstimulation. Front Integr Neurosci 2009; 3: 20, https://doi.org/10.3389/neuro.07.020.2009.
- Fitzsimmons N.A., Lebedev M.A., Peikon I.D., Nicolelis M.A.L. Extracting kinematic parameters for monkey bipedal walking from cortical neuronal ensemble activity. Front Integr Neurosci 2009; 3: 3, https://doi.org/10.3389/neuro.07.003.2009.
- Velliste M., Perel S., Spalding M.C., Whitford A.S., Schwartz A.B. Cortical control of a prosthetic arm for self-feeding. Nature 2008; 453(7198): 1098–1101, https://doi.org/10.1038/nature06996.
- Capogrosso M., Milekovic T., Borton D., Wagner F., Moraud E.M., Mignardot J.B., Buse N., Gandar J., Barraud Q., Xing D., Rey E., Duis S., Jianzhong Y., Ko W.K., Li Q., Detemple P., Denison T., Micera S., Bezard E., Bloch J., Courtine G. A brain-spine interface alleviating gait deficits after spinal cord injury in primates. Nature 2016; 539(7628): 284–288, https://doi.org/10.1038/nature20118.
- Ganguly K., Carmena J.M. Emergence of a stable cortical map for neuroprosthetic control. PLoS Biol 2009; 7(7): e1000153, https://doi.org/10.1371/journal.pbio.1000153.
- Gilja V., Nuyujukian P., Chestek C.A., Cunningham J.P., Yu B.M., Fan J.M., Churchland M.M., Kaufman M.T., Kao J.C., Ryu S.I., Shenoy K.V. A high-performance neural prosthesis enabled by control algorithm design. Nat Neurosci 2012; 15(12): 1752–1757, https://doi.org/10.1038/nn.3265.
- Ma X., Rizzoglio F., Bodkin K.L., Perreault E., Miller L.E., Kennedy A. Using adversarial networks to extend brain computer interface decoding accuracy over time. ELife 2023; 12: e84296, https://doi.org/10.7554/elife.84296.
- Athalye V.R., Khanna P., Gowda S., Orsborn A.L., Costa R.M., Carmena J.M. Invariant neural dynamics drive commands to control different movements. Curr Biol 2023; 33(14): 2962–2976.e15, https://doi.org/10.1016/j.cub.2023.06.027.
- Borra D., Filippini M., Ursino M., Fattori P., Magosso E. Motor decoding from the posterior parietal cortex using deep neural networks. J Neural Eng 2023; 20(3): 036016, https://doi.org/10.1088/1741-2552/acd1b6.
- Öztürk S., Devecioğlu İ., Güçlü B. Bayesian prediction of psychophysical detection responses from spike activity in the rat sensorimotor cortex. J Comput Neurosci 2023; 51(2): 207–222, https://doi.org/10.1007/s10827-023-00844-0.
- Orsborn A.L., Moorman H.G., Overduin S.A., Shanechi M.M., Dimitrov D.F., Carmena J.M. Closed-loop decoder adaptation shapes neural plasticity for skillful neuroprosthetic control. Neuron 2014; 82(6): 1380–1393, https://doi.org/10.1016/j.neuron.2014.04.048.
- Kao J.C., Nuyujukian P., Ryu S.I., Shenoy K.V. A high-performance neural prosthesis incorporating discrete state selection with hidden Markov models. IEEE Trans Biomed Eng 2017; 64(4): 935–945, https://doi.org/10.1109/tbme.2016.2582691.
- Willsey M.S., Nason-Tomaszewski S.R., Ensel S.R., Temmar H., Mender M.J., Costello J.T., Patil P.G., Chestek C.A. Real-time brain–machine interface in non-human primates achieves high-velocity prosthetic finger movements using a shallow feedforward neural network decoder. Nat Commun 2022; 13(1): 6899, https://doi.org/10.1038/s41467-022-34452-w.
- Левицкая О.С., Лебедев М.А. Интерфейс мозг–компьютер: будущее в настоящем. Вестник Российского государственного медицинского университета 2016; 2: 4–16, https://doi.org/10.24075/brsmu.2016-02-01.
- Dennett D.C. A treat: Grey Walter’s precognitive carousel. In: Consciousness explained. New York, Boston, London: Back Bay Books/Little, Brown and Company, Hachette Book Group USA; 1991; p. 167–168.
- Kennedy P.R., Bakay R.A. Restoration of neural output from a paralyzed patient by a direct brain connection. Neuroreport 1998; 9(8): 1707–1711, https://doi.org/10.1097/00001756-199806010-00007.
- Kennedy P.R., Bakay R.A.E., Moore M.M., Adams K., Goldwaithe J. Direct control of a computer from the human central nervous system. IEEE Trans Rehabil Eng 2000; 8(2): 198–202, https://doi.org/10.1109/86.847815.
- Kennedy P.R., Kirby M.T., Moore M.M., King B., Mallory A. Computer control using human intracortical local field potentials. IEEE Trans Neural Syst Rehabil Eng 2004; 12(3): 339–344, https://doi.org/10.1109/tnsre.2004.834629.
- Bartels J., Andreasen D., Ehirim P., Mao H., Seibert S., Wright E.J., Kennedy P. Neurotrophic electrode: method of assembly and implantation into human motor speech cortex. J Neurosci Methods 2008; 174(2): 168–176, https://doi.org/10.1016/j.jneumeth.2008.06.030.
- Guenther F.H., Brumberg J.S., Wright E.J., Nieto-Castanon A., Tourville J.A., Panko M., Law R., Siebert S.A., Bartels J.L., Andreasen D.S., Ehirim P., Mao H., Kennedy P.R. A wireless brain-machine interface for real-time speech synthesis. PloS One 2009; 4(12): e8218, https://doi.org/10.1371/journal.pone.0008218.
- Brumberg J.S., Nieto-Castanon A., Kennedy P.R., Guenther F.H. Brain–computer interfaces for speech communication. Speech Commun 2010; 52(4): 367–379, https://doi.org/10.1016/j.specom.2010.01.001.
- Лебедев М.А. Нейрокомпьютерные интерфейсы для расширения функций мозга. Наука и инновации в медицине 2016; 1(3): 11–27, https://doi.org/10.35693/2500-1388-2016-0-3-11-27.
- Maynard E.M., Nordhausen C.T., Normann R.A. The Utah intracortical electrode array: a recording structure for potential brain-computer interfaces. Electroencephalogr Clin Neurophysiol 1997; 102(3): 228–239, https://doi.org/10.1016/s0013-4694(96)95176-0.
- Guillory K.S., Normann R.A. A 100-channel system for real time detection and storage of extracellular spike waveforms. J Neurosci Methods 1999; 91(1–2): 21–29, https://doi.org/10.1016/s0165-0270(99)00076-x.
- Suner S., Fellows M.R., Vargas-Irwin C., Nakata G.K., Donoghue J.P. Reliability of signals from a chronically implanted, silicon-based electrode array in non-human primate primary motor cortex. IEEE Trans Neural Syst Rehabil Eng 2005; 13(4): 524–541, https://doi.org/10.1109/tnsre.2005.857687.
- Serruya M.D., Hatsopoulos N.G., Paninski L., Fellows M.R., Donoghue J.P. Instant neural control of a movement signal. Nature 2002; 416(6877): 141–142, https://doi.org/10.1038/416141a.
- Donoghue J.P., Nurmikko A., Black M., Hochberg L.R. Assistive technology and robotic control using motor cortex ensemble-based neural interface systems in humans with tetraplegia. J Physiol 2007; 579(Pt 3): 603–611, https://doi.org/10.1113/jphysiol.2006.127209.
- Kim S.P., Simeral J.D., Hochberg L.R., Donoghue J.P., Friehs G.M., Black M.J. Point-and-click cursor control with an intracortical neural interface system by humans with tetraplegia. IEEE Trans Neural Syst Rehabil Eng 2011; 19(2): 193–203, https://doi.org/10.1109/tnsre.2011.2107750.
- Truccolo W., Friehs G.M., Donoghue J.P., Hochberg L.R. Primary motor cortex tuning to intended movement kinematics in humans with tetraplegia. J Neurosci 2008; 28(5): 1163–1178, https://doi.org/10.1523/jneurosci.4415-07.2008.
- Kim S.P., Simeral J.D., Hochberg L.R., Donoghue J.P., Black M.J. Neural control of computer cursor velocity by decoding motor cortical spiking activity in humans with tetraplegia. J Neural Eng 2008; 5(4): 455–476, https://doi.org/10.1088/1741-2560/5/4/010.
- Simeral J.D., Kim S.P., Black M.J., Donoghue J.P., Hochberg L.R. Neural control of cursor trajectory and click by a human with tetraplegia 1000 days after implant of an intracortical microelectrode array. J Neural Eng 2011; 8(2): 025027, https://doi.org/10.1088/1741-2560/8/2/025027.
- Wodlinger B., Downey J.E., Tyler-Kabara E.C., Schwartz A.B., Boninger M.L., Collinger J.L. Ten-dimensional anthropomorphic arm control in a human brain-machine interface: difficulties, solutions, and limitations. J Neural Eng 2015; 12(1): 016011, https://doi.org/10.1088/1741-2560/12/1/016011.
- Downey J.E., Brane L., Gaunt RA., Tyler-Kabara EC., Boninger M.L., Collinger J.L. Motor cortical activity changes during neuroprosthetic-controlled object interaction. Sci Rep 2017; 7(1): 16947, https://doi.org/10.1038/s41598-017-17222-3.
- Handelman D.A., Osborn L.E., Thomas T.M., Badger A.R., Thompson M., Nickl R.W., Anaya M.A., Wormley J.M., Cantarero G.L., McMullen D., Crone N.E., Wester B., Celnik P.A., Fifer M.S., Tenore F.V. Shared control of bimanual robotic limbs with a brain-machine interface for self-feeding. Front Neurorobot 2022; 16: 918001, https://doi.org/10.3389/fnbot.2022.918001.
- Gilja V., Pandarinath C., Blabe C.H., Nuyujukian P., Simeral J.D., Sarma A.A., Sorice B.L., Perge J.A., Jarosiewicz B., Hochberg L.R., Shenoy K.V., Henderson J.M. Clinical translation of a high-performance neural prosthesis. Nat Med 2015; 21(10): 1142–1145, https://doi.org/10.1038/nm.3953.
- Brandman D.M., Hosman T., Saab J., Burkhart M.C., Shanahan B.E., Ciancibello J.G., Sarma A.A., Milstein D.J., Vargas-Irwin C.E., Franco B., Kelemen J., Blabe C., Murphy B.A., Young D.R., Willett F.R., Pandarinath C., Stavisky S.D., Kirsch R.F., Walter B.L., Bolu Ajiboye A., Cash S.S., Eskandar E.N., Miller J.P., Sweet J.A., Shenoy K.V., Henderson J.M., Jarosiewicz B., Harrison M.T., Simeral J.D., Hochberg L.R. Rapid calibration of an intracortical brain-computer interface for people with tetraplegia. J Neural Eng 2018; 15(2): 026007, https://doi.org/10.1088/1741-2552/aa9ee7.
- Paulo D.L., Sheth S.A., McKhann G.M. II. Living brain to hand: bypassing spinal cord quadriplegia using a novel neuroprosthesis. Neurosurgery 2016; 79(2): N13–N14, https://doi.org/10.1227/01.neu.0000489885.18088.eb.
- Chadwick E.K., Blana D., Simeral J.D., Lambrecht J., Kim S.P., Cornwell A.S., Taylor D.M., Hochberg L.R., Donoghue J.P., Kirsch R.F. Continuous neuronal ensemble control of simulated arm reaching by a human with tetraplegia. J Neural Eng 2011; 8(3): 034003, https://doi.org/10.1088/1741-2560/8/3/034003.
- Bouton C.E., Shaikhouni A., Annetta N.V., Bockbrader M.A., Friedenberg D.A., Nielson D.M., Sharma G., Sederberg P.B., Glenn B.C., Mysiw W.J., Morgan A.G., Deogaonkar M., Rezai A.R. Restoring cortical control of functional movement in a human with quadriplegia. Nature 2016; 533(7602): 247–250, https://doi.org/10.1038/nature17435.
- Hughes C., Herrera A., Gaunt R., Collinger J. Bidirectional brain-computer interfaces. Handb Clin Neurol 2020; 168: 163–181, https://doi.org/10.1016/B978-0-444-63934-9.00013-5.
- Bacher D., Jarosiewicz B., Masse N.Y., Stavisky S.D., Simeral J.D., Newell K., Oakley E.M., Cash S.S., Friehs G., Hochberg L.R. Neural point-and-click communication by a person with incomplete locked-in syndrome. Neurorehabil Neural Repair 2015; 29(5): 462–471, https://doi.org/10.1177/1545968314554624.
- Jarosiewicz B., Sarma A.A., Bacher D., Masse N.Y., Simeral J.D., Sorice B., Oakley E.M., Blabe C., Pandarinath C., Gilja V., Cash S.S., Eskandar E.N., Friehs G., Henderson J.M., Shenoy K.V., Donoghue J.P., Hochberg L.R. Virtual typing by people with tetraplegia using a self-calibrating intracortical brain-computer interface. Sci Transl Med 2015; 7(313): 313ra179, https://doi.org/10.1126/scitranslmed.aac7328.
- Pandarinath C., Nuyujukian P., Blabe C.H., Sorice B.L., Saab J., Willett F.R., Hochberg L.R., Shenoy K.V., Henderson J.M. High performance communication by people with paralysis using an intracortical brain-computer interface. ELife 2017; 6: e18554, https://doi.org/10.7554/elife.18554.
- Nuyujukian P., Albites Sanabria J., Saab J., Pandarinath C., Jarosiewicz B., Blabe C.H., Franco B., Mernoff S.T., Eskandar E.N., Simeral J.D., Hochberg L.R., Shenoy K.V., Henderson J.M. Cortical control of a tablet computer by people with paralysis. PloS One 2018; 13(11): e0204566, https://doi.org/10.1371/journal.pone.0204566.
- Simeral J.D., Hosman T., Saab J., Flesher S.N., Vilela M., Franco B., Kelemen J.N., Brandman D.M., Ciancibello J.G., Rezaii P.G., Eskandar E.N., Rosler D.M., Shenoy K.V., Henderson J.M., Nurmikko A.V., Hochberg L.R. Home use of a percutaneous wireless intracortical brain-computer interface by individuals with tetraplegia. IEEE Trans Biomed Eng 2021; 68(7): 2313–2325, https://doi.org/10.1109/tbme.2021.3069119.
- Shah N.P., Willsey M.S., Hahn N., Kamdar F., Avansino D.T., Hochberg L.R., Shenoy K.V., Henderson J.M. A brain-computer typing interface using finger movements. Int IEEE EMBS Conf Neural Eng 2023; 2023: 10.1109/ner52421.2023.10123912, https://doi.org/10.1109/ner52421.2023.10123912.
- Dekleva B.M., Weiss J.M., Boninger M.L., Collinger J.L. Generalizable cursor click decoding using grasp-related neural transients. J Neural Eng 2021; 18(4): 0460e9, https://doi.org/10.1088/1741-2552/ac16b2.
- Jun J.J., Steinmetz N.A., Siegle J.H., Denman D.J., Bauza M., Barbarits B., Lee A.K., Anastassiou C.A., Andrei A., Aydın Ç., Barbic M., Blanche T.J., Bonin V., Couto J., Dutta B., Gratiy S.L., Gutnisky D.A., Häusser M., Karsh B., Ledochowitsch P., Lopez C.M., Mitelut C., Musa S., Okun M., Pachitariu M., Putzeys J., Rich P.D., Rossant C., Sun W.L., Svoboda K., Carandini M., Harris K.D., Koch C., O’Keefe J., Harris T.D. Fully integrated silicon probes for high-density recording of neural activity. Nature 2017; 551(7679): 232–236, https://doi.org/10.1038/nature24636.
- Musk E.; Neuralink. An integrated brain-machine interface platform with thousands of channels. J Med Internet Res 2019; 21(10): e16194, https://doi.org/10.2196/16194.
- Nurmikko A. Challenges for large-scale cortical interfaces. Neuron 2020; 108(2): 259–269, https://doi.org/10.1016/j.neuron.2020.10.015.
- Vázquez-Guardado A., Yang Y., Bandodkar A.J., Rogers J.A. Recent advances in neurotechnologies with broad potential for neuroscience research. Nat Neurosci 2020; 23(12): 1522–1536, https://doi.org/10.1038/s41593-020-00739-8.
- Sahasrabuddhe K., Khan A.A., Singh A.P., Stern T.M., Ng Y., Tadić A., Orel P., LaReau C., Pouzzner D., Nishimura K., Boergens K.M., Shivakumar S., Hopper M.S., Kerr B., Hanna M.E.S., Edgington R.J., McNamara I., Fell D., Gao P., Babaie-Fishani A., Veijalainen S., Klekachev A.V., Stuckey A.M., Luyssaert B., Kozai T.D.Y., Xie C., Gilja V., Dierickx B., Kong Y., Straka M., Sohal H.S., Angle M.R. The Argo: a 65,536 channel recording system for high density neural recording in vivo. BioRxiv; 2020, https://doi.org/10.1101/2020.07.17.209403.
- Zhou Y., Yang H., Wang X., Yang H., Sun K., Zhou Z., Sun L., Zhao J., Tao T.H., Wei X. A mosquito mouthpart-like bionic neural probe. Microsyst Nanoeng 2023; 9: 88, https://doi.org/10.1038/s41378-023-00565-5.
- Trotier A., Bagnoli E., Walski T., Evers J., Pugliese E., Lowery M., Kilcoyne M., Fitzgerald U., Biggs M. Micromotion derived fluid shear stress mediates peri-electrode gliosis through mechanosensitive ion channels. Adv Sci (Weinh) 2023; 10(27): e2301352, https://doi.org/10.1002/advs.202301352.
- Petrosyan A., Voskoboinikov A., Sukhinin D., Makarova A., Skalnaya A., Arkhipova N., Sinkin M., Ossadtchi A. Speech decoding from a small set of spatially segregated minimally invasive intracranial EEG electrodes with a compact and interpretable neural network. J Neural Eng 2022; 19(6): 066016, https://doi.org/10.1088/1741-2552/aca1e1.
- Chen K., Cambi F., Kozai T.D.Y. Pro-myelinating clemastine administration improves recording performance of chronically implanted microelectrodes and nearby neuronal health. Biomaterials 2023; 301: 122210, https://doi.org/10.1016/j.biomaterials.2023.122210.
- Bergeron D., Iorio-Morin C., Bonizzato M., Lajoie G., Orr Gaucher N., Racine É., Weil A.G. Use of invasive brain-computer interfaces in pediatric neurosurgery: technical and ethical considerations. J Child Neurol 2023; 38(3–4): 223–238, https://doi.org/10.1177/08830738231167736.
- Nowik K., Langwińska-Wośko E., Skopiński P., Nowik K.E., Szaflik J.P. Bionic eye review — an update. J Clin Neurosci 2020; 78: 8–19, https://doi.org/10.1016/j.jocn.2020.05.041.
- Drew L. The rise of brain-reading technology: what you need to know. Nature 2023; 623(7986): 241–243, https://doi.org/10.1038/d41586-023-03423-6.